What does intense heat, penetrating deeply, signify? Understanding the implications of profound and intense thermal energy.
This describes a significant degree of heat that extends to a substantial depth. Think of the intense, pervasive warmth experienced within a geothermal vent, or the deep internal heating of a material during high-temperature industrial processes. The effect is characterized by a high thermal gradient, where temperature changes drastically over a relatively short distance. This contrasts with superficial heating, where heat remains concentrated near the surface.
The significance of such profound heat depends entirely on the context. In geological settings, it reveals the internal workings of the planet, possibly indicating volcanic activity or the presence of geothermal resources. In industrial applications, it's a critical factor in metallurgy, materials processing, and various other manufacturing operations, directly impacting the quality and properties of finished products. The precise implications will vary greatly from one scenario to another.
Moving forward, a detailed exploration of various applications will illustrate how the principles of deep heat, or intense internal heating, play a crucial role in specific industrial processes. Understanding these processes and their effects is essential for developing effective safety measures, optimizing production, and managing resources efficiently.
Deep Hot
Understanding the nuances of "deep hot" requires examining its inherent qualities as both an adjective and a descriptive term. This analysis considers various dimensions of intense heat and its effects.
- Thermal intensity
- Penetration depth
- Material impact
- Geothermal processes
- Industrial applications
- Safety considerations
These aspects, while seemingly disparate, are interconnected. Thermal intensity, for example, directly relates to penetration depth, influencing the material impact. Deep geothermal processes, often characterized by profound heat, have implications for industrial applications, while safety protocols are paramount to mitigating any hazards. Consider the heat treatment of metals; high temperatures are applied deeply to transform the metal's structure, demonstrating the material impact. Understanding these interconnected aspects helps illustrate how "deep hot" conditions play a critical role in both natural and engineered environments.
1. Thermal Intensity
Thermal intensity forms a crucial component of "deep hot" conditions. High thermal intensity is characterized by a significant amount of heat energy, capable of penetrating deeply into materials and systems. This profound heat often necessitates careful consideration of its effects, which vary based on the nature of the material and the specific application.
- Relationship to Penetration Depth
Thermal intensity directly influences the depth to which heat penetrates. Higher intensity generally allows for deeper penetration, leading to a wider impact on the material's properties and structure. This deeper penetration is a key characteristic of "deep hot" conditions. Examples include geothermal processes, where intense heat penetrates deeply into the Earth's crust, or specialized industrial heating procedures targeting specific internal structural transformations in metals.
- Impact on Material Properties
The intensity of heat directly affects the changes within materials. High thermal intensity can induce significant alterations in material structure, phase transitions, and chemical reactions. This is especially relevant for manufacturing processes, where controlling thermal intensity is critical for producing desired material properties. Examples include heat treatments used in steel production to achieve specific hardness and ductility.
- Scaling Considerations
The level of thermal intensity must be considered in relation to the scale of the system. In smaller-scale applications, high thermal intensity might be achievable and manageable, while larger-scale systems may demand meticulous control and specialized infrastructure to achieve the same degree of deep heating. This scaling issue impacts the engineering and safety aspects of applying intense heat. For instance, heating a small metal ingot differs considerably from heating a large furnace for industrial production.
- Consequences of Inappropriate Intensity Control
Insufficient or excessive thermal intensity can lead to undesirable outcomes. Under-heating may result in incomplete transformation or alteration of the target material; over-heating could cause damage, structural failure, or unwanted chemical reactions. The consequences can range from minor quality issues in manufacturing to significant safety hazards in industrial settings.
In conclusion, thermal intensity is inextricably linked to "deep hot" conditions. Understanding its relationship to penetration depth, material impact, scaling considerations, and potential consequences is fundamental for effective applications and responsible management of such intense heat.
2. Penetration Depth
Penetration depth, a crucial aspect of "deep hot" conditions, refers to the extent to which heat penetrates into a material. This depth is directly influenced by the intensity of the heat source and the material's properties. A high penetration depth is characteristic of "deep hot" situations, indicating that the heat affects a significant volume of material rather than just a superficial layer. The importance of penetration depth stems from its impact on material transformation, structural changes, and overall performance.
Several factors contribute to penetration depth. Heat conductivity of the material is paramount; highly conductive materials allow heat to penetrate more readily than those with lower conductivity. The duration of heat application also plays a significant role; prolonged exposure allows for greater penetration. Furthermore, the geometry of the heat source and the material itself influences the way heat propagates. For instance, focused laser beams can achieve high penetration depths in specific areas, while large-scale furnaces distribute heat over a broader area with potentially shallower penetration. Understanding these interdependencies is critical for controlling the effects of heat on various materials and systems.
Real-world examples illustrate the practical significance of penetration depth. In forging, the penetration depth of the heat determines the degree to which the metal's internal structure is altered, impacting the final product's strength and ductility. In heat treatments for metals, precise control of penetration depth is essential to achieving the desired material properties. Deep penetration is vital in many industrial processes, including casting, welding, and annealing, ensuring uniform heating and structural modifications throughout the material. Failure to consider penetration depth can lead to inconsistencies in the treated materials, compromising their overall quality and potentially increasing the risk of failure.
In summary, penetration depth is a fundamental parameter in "deep hot" processes. Its interplay with heat intensity, material properties, and application duration directly impacts material transformation and overall performance. Thorough understanding and precise control of penetration depth are essential for achieving desired outcomes in a wide range of industrial applications, demanding meticulous attention to detail and advanced analysis to ensure consistency and efficacy.
3. Material Impact
Material impact, within the context of "deep hot" conditions, encompasses the profound effects intense heat has on the physical and chemical properties of materials. This includes alterations in structure, phase transitions, and chemical reactions. The severity and nature of these impacts are directly correlated to the degree of heat penetration and the material's inherent characteristics. Crucially, understanding these impacts is vital for effective material processing and utilization.
The interplay between intense heat and material properties is a complex one. High-temperature exposure can induce recrystallization, altering the grain structure and consequently impacting mechanical properties such as strength and ductility. Phase transitions, like melting or the transformation from one crystalline structure to another, are common responses to deep heating, fundamentally changing a material's characteristics. Chemical reactions, sometimes accelerated by high temperatures, can also alter the material's composition and properties. For example, carbon can diffuse into steel during high-temperature heat treatments, impacting its hardness. Precise control over these transformations is essential for attaining desired material properties in applications like manufacturing and engineering.
Understanding the material impact of "deep hot" conditions is crucial for several reasons. Foremost, it allows for the prediction and control of material behavior under extreme heat conditions. This predictive capability is critical for designing systems and processes that can withstand these conditions without structural failure. In metallurgical applications, predicting and controlling material behavior during forging, casting, and heat treatment is crucial for achieving desired material properties. Moreover, this understanding is vital for preventing unintended consequences, such as material degradation, failure, or unsafe operational conditions. Examples include ensuring the structural integrity of components in high-temperature environments, such as jet engines, or mitigating potential damage from accidental overheating. Finally, the ability to manipulate material behavior through targeted heating is crucial for the development and improvement of advanced materials with specific tailored properties for diverse applications, from aerospace engineering to medical implants.
4. Geothermal Processes
Geothermal processes inherently involve deep, intense heat originating from the Earth's interior. This deep heat, often at extreme temperatures, is a defining characteristic of these processes and directly relates to the concept of "deep hot." Understanding these processes provides valuable insights into Earth's internal workings and resource potential.
- Heat Source and Generation
Geothermal energy derives from the Earth's internal heat, a legacy of planetary formation and ongoing radioactive decay within the Earth's mantle. This heat energy is transferred through various geological mechanisms, driving hydrothermal convection and creating gradients of temperature within the Earth's crust. This internal heat source is fundamental to geothermal processes, creating the "deep hot" conditions necessary for resource extraction and geological phenomena. Different geothermal systems exhibit varying heat intensities depending on the source and depth of the heat generation.
- Hydrothermal Systems and Features
Deep hot environments, driven by geothermal activity, often form hydrothermal systems. These systems involve circulating water or other fluids heated by underlying magma or hot rock. The resulting hot fluids may emerge as geysers, hot springs, or fumaroles, showcasing the intense, deep heat manifesting at the Earth's surface. The formation and characteristics of these features depend on the specific geological context, highlighting the complex interplay between deep-seated heat and surface expressions.
- Geological Implications and Applications
Geothermal processes significantly influence geological features and the composition of the Earth's crust. Examples include the formation of specific rock types through metamorphism, mineral deposition, and the alteration of existing rock formations. These processes are also relevant to the search for resources like geothermal energy, which harnesses the Earth's internal heat for power generation, and identifying areas with potential for geothermal energy extraction.
- Environmental Impacts
Geothermal activity can have various environmental impacts, ranging from localized effects like altered landscapes and geochemical changes to broader consequences like the release of gases. The precise effects depend on the intensity and scale of the geothermal process, necessitating careful environmental assessment before exploiting geothermal resources. Considerations of ground subsidence, gas emissions, and potential for changes in the local hydrology are crucial components of understanding these implications.
In conclusion, geothermal processes exemplify "deep hot" conditions by manifesting Earth's internal heat at varying scales and depths. Understanding the interplay of heat sources, hydrothermal systems, geological influences, and potential environmental impacts is vital for responsible resource management and informed geological analysis.
5. Industrial Applications
Industrial applications are intrinsically linked to "deep hot" conditions. Intense heat is crucial for numerous manufacturing processes, transforming raw materials into finished products. The application of deep heat influences material properties, enabling desired outcomes in diverse industries. This necessitates careful control and management of heat sources and intensities. Understanding this connection is vital for optimizing production, ensuring safety, and developing innovative processes.
Specific industrial applications often require precisely controlled high temperatures for transformative effects. Metallurgy, for instance, employs various heat treatments to alter the microstructure of metals, enhancing their strength, hardness, and ductility. Forging, casting, and heat treatments depend on the ability to generate and manage deep hot conditions. The semiconductor industry also relies on "deep hot" processes to create integrated circuits, impacting electronics production and related fields. Furthermore, chemical synthesis often necessitates high temperatures and pressures to achieve specific reactions, producing critical materials for various industrial sectors. In these contexts, controlling deep heat and understanding its consequences are fundamental to success.
The understanding of "deep hot" in industrial applications holds practical significance across diverse sectors. This knowledge is essential for process optimization, as it allows for the precise control of material transformations. Optimizing energy consumption becomes possible when the interplay between heat input and material response is well understood. Furthermore, this understanding underpins the development of innovative processes, leading to enhanced product properties. Crucially, it informs safety protocols for handling high-temperature operations, mitigating potential hazards. For example, accurate thermal modeling allows for the design of robust equipment capable of withstanding intense heat, preventing malfunctions and ensuring operational safety in industries like power generation and metallurgy.
6. Safety Considerations
Safety considerations are paramount when dealing with "deep hot" conditions. The inherent risks associated with extreme temperatures necessitate proactive measures to prevent accidents and ensure the well-being of personnel and the integrity of equipment. The connection between these factors is direct and crucial, impacting all stages from process design to operational management. Failure to account for safety implications can lead to catastrophic consequences, underscoring the importance of a robust safety framework within any operation involving intense heat.
A comprehensive safety framework encompassing "deep hot" operations must address potential hazards at various levels. Equipment design must incorporate thermal shielding, insulation, and robust materials capable of withstanding extreme temperatures, preventing material failure and consequent accidents. Monitoring systems are vital for continuous temperature surveillance and immediate alerts for deviations from safe operating parameters. Emergency shutdown mechanisms and protocols must be readily available and functional, allowing for rapid response to unforeseen events. Personnel training is critical, ensuring workers understand the potential hazards and proper procedures for operating in high-temperature environments. Clear communication channels and well-defined emergency response plans are indispensable. Furthermore, regular inspections and maintenance are essential to verify the continued integrity of equipment and systems. Real-life examples underscore the need for thorough safety protocols; accidents involving catastrophic equipment failures often stem from inadequate safety measures or ignored warnings. These incidents highlight the direct link between safety negligence and severe consequences.
In conclusion, safety considerations are not an add-on but a fundamental component of any operation involving "deep hot" conditions. The necessity for comprehensive safety protocols is unequivocal. Failure to prioritize safety in these contexts can result in significant losses, injuries, and damage, highlighting the critical role of proactive safety measures in mitigating potential risks and ensuring the well-being of personnel and the integrity of facilities. A profound understanding of the interrelation between safety measures and "deep hot" applications is paramount for preventing catastrophic incidents and optimizing operations.
Frequently Asked Questions
This section addresses common inquiries regarding "deep hot" conditions, encompassing intense heat and its implications across various contexts. Clear and concise answers are provided to foster a comprehensive understanding.
Question 1: What distinguishes "deep hot" from other forms of high-temperature environments?
Answer 1: "Deep hot" conditions are characterized by intense heat penetrating to significant depths within a material or system. This contrasts with surface heating, where heat remains concentrated near the exterior. The crucial distinction lies in the extent of thermal penetration, impacting material properties and overall behavior.
Question 2: What factors influence the penetration depth of intense heat?
Answer 2: Several factors govern heat penetration depth. Material conductivity plays a crucial role; highly conductive materials permit deeper penetration. The duration of heat exposure also significantly impacts penetration, with prolonged exposure facilitating deeper penetration. The configuration of the heat source and the target material's geometry influence how heat propagates.
Question 3: What are the implications of "deep hot" on material properties?
Answer 3: Intense heat can induce structural alterations, phase transitions, and chemical reactions within materials. These effects can impact mechanical properties like strength and ductility, modify phase compositions, and accelerate chemical transformations within the target material. The degree and type of changes depend on the specific material and the intensity of the heat.
Question 4: How is "deep hot" applied in industrial settings?
Answer 4: "Deep hot" conditions are essential in various industrial processes, including metallurgy (heat treatments), semiconductor manufacturing, and chemical synthesis. These processes often require precise control of both temperature and penetration depth to achieve desired material properties and product specifications. Controlling the heat input and its effect is vital to these industrial applications.
Question 5: What safety considerations are crucial for working with "deep hot" environments?
Answer 5: Safety is paramount in "deep hot" environments. Robust equipment design, thermal shielding, continuous monitoring, and emergency protocols are essential. Adequate training and clear communication protocols for personnel handling such conditions are vital to mitigating risks. Regular inspections and maintenance are equally crucial to preventing accidents and safeguarding workers.
In summary, understanding "deep hot" conditions requires analyzing the interplay of heat intensity, penetration depth, material impact, and safety implications. This comprehensive perspective is vital for both industrial applications and scientific investigations of profound thermal energy.
The subsequent sections delve deeper into specific applications and methodologies for managing and utilizing "deep hot" conditions.
Conclusion
This exploration of "deep hot" conditions has illuminated the multifaceted nature of intense, deeply penetrating heat. Key aspects examined include the profound influence of thermal intensity on penetration depth and resulting material impacts. Geothermal processes, demonstrating natural occurrences of deep heat, provide a crucial context for understanding the industrial applications of controlled high-temperature environments. Safety considerations, integral to any operation involving such extreme temperatures, were emphasized as crucial for preventing accidents. The analysis highlighted the intricate relationship between heat intensity, penetration depth, and the subsequent transformation of materials. This interplay underscores the importance of meticulous control and precise understanding of these factors for achieving desired outcomes in diverse industrial settings.
Moving forward, continued research into "deep hot" phenomena remains crucial. Further investigation into the complex interactions between heat, materials, and systems can lead to breakthroughs in diverse fields, from developing advanced materials to enhancing energy efficiency. The intricate interplay of physical and chemical processes driven by deep heat demands sustained attention, fostering innovation, safety, and responsible resource management. Understanding and mastering "deep hot" conditions is not just a matter of technological advancement but a cornerstone for addressing global challenges in energy, materials science, and environmental sustainability.
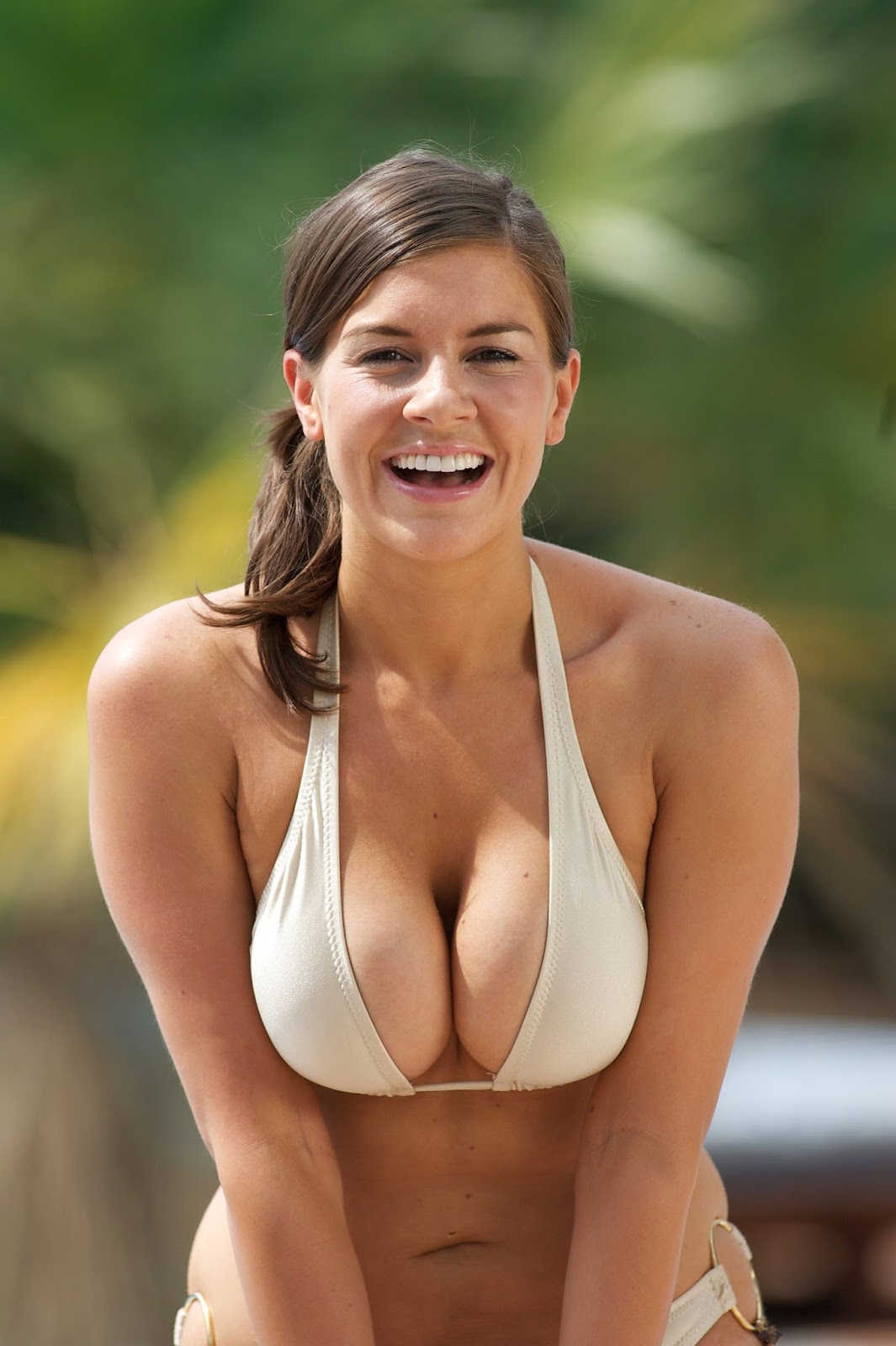

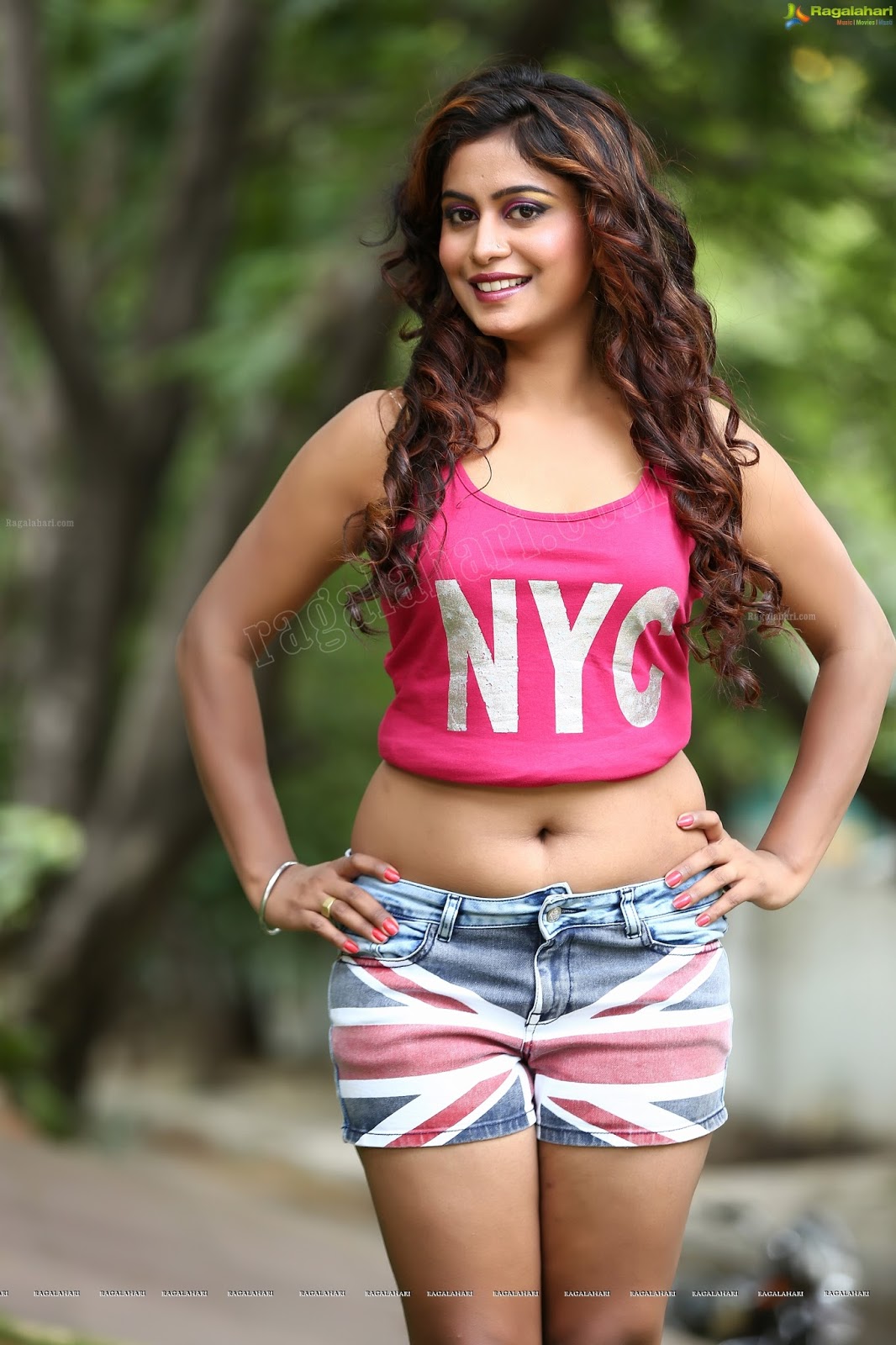